The Ultimate Guide to Ion Propulsion
- Prof.Smith
- Sep 3, 2019
- 11 min read
Updated: Feb 27, 2023
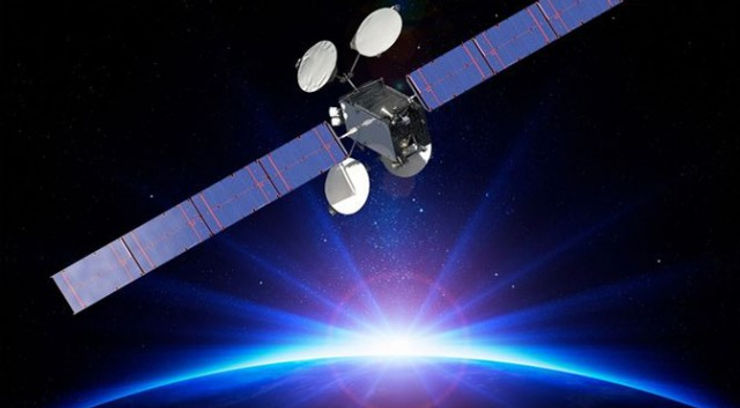
Since the very beginnings of the 20th century, American Physicist Robert Goddard noticed the possibility of accelerating electrically charged particles to very high velocities without the need for high temperatures. The term ’ion propulsion’ today refers to an engine which accelerates ions at very high speeds, (using a voltage difference). According to Newton’s third law this creates a ‘thrust’ towards the opposite direction. These ‘ion thrusters’ are an important novelty in space technology, with many advantages over conventional chemical rockets. An ion thruster relies on the same simple equation as any other rocket engine. The thrust (F) it produces is equal to the mass (m) of fuel moved times its acceleration (a). Thus F = ma. While conventional chemical rockets accelerate their fuels at 6,000 miles per hour, achieving more thrust requires more propellant, however accelerating that propellant requires even more propellant. An ion thruster's solution to this unpleasant cycle of adding too much mass to the spaceship, as it uses electricity to accelerate ions.
It can accelerate ions at 60,000 miles per hour, thus achieving high thrust, with much less fuel. The biggest novelty of an ion thruster is its source of energy. Chemical rockets store energy in the chemicals, but ion thrusters work with electric energy, which can be taken from solar cells, which make the electricity for the electrostatic field that moves the ions. It is important to note, that the thruster will push the spaceship as hard as a piece of paper pushes a human hand. It will take one whole day to accelerate the craft as fast as it would accelerate after dropping in Earth's gravity for one second.
After a few months of operation, that tiny force will speed the craft up by 8,000 mph. Spending only a few milligrams of fuel per second ion engines can operate over much longer periods of time than chemical rockets. However, ion propulsion cannot be used to launch a spacecraft from the earth’s surface; the secret is in the ability of ion space crafts to accumulate acceleration over months of ejecting ions. For missions outside the asteroid belt a nuclear power source, might be a better option (solar energy becomes weak enough at some point). An important aim of this article is to uncover the advantages of ion propulsion over the ordinary engines, using liquid fuels.
The modern history of rockets starts at the early 20th century, with the famous attempts of the American physicist Robert Goddard, to launch rockets containing liquid fuels and oxygen. This was an innovative idea at the time, and many people thought he was mad. However even as early as 300BC Gunpowder-filled bamboo tubes were used for fireworks in China. Almost 1000 years later we can witness the use of military rockets in China. However nobody could imagine how would be possible to make a rocket move in space. In 1895 Konstantin Tsiolkovsky, set the foundations of rocket theory by deriving the fundamental rocket equation. Goddard, applied that theory. He launched the first model rocket of a new age in 1926. Old, modern, and at least, the vast majority of science fiction rockets rely on the very elementary Newton’s third law. Chemical rockets were used in world War II by Wernher von Braun's team, and 15 years later, they were used to launch the first Earth’s satellite. Today of course the launch of chemical rockets is a matter of routine.
A new page, in the history of space exploration and spaceships, is ion propulsion. The theoretical discussion that follows helps us to get an idea of how the principles of ion propulsion initiated and evolved and it prepares the ground for a more interesting development of the physics of ion propulsion. In 1906 Robert Goddard mentioned the possibility of accelerating electrically charged particles to very high velocities without the need for high temperatures. In Early 1920 Werner von Braun and Dr. Hermann Oberth considered seriously the possibility of electronic propulsion. Oberth proposed an “electric rocket.”In his book ‘Possibilities of Space Flight’ which was published in 1939, Oberth devoted a chapter to electric propulsion. In 1947 Werner von Braun told Stuhlinger: "Professor Oberth has been right with so many of his early proposals, I wouldn’t be a bit surprised if we flew to Mars electrically.”
11 years later Stuhlinger presented a paper at the International Congress in Vienna with the title: “Possibilities of Electrical Space Ship Propulsion.” During his presentation, Stuhlinger discussed a proposal made by von Braun two years earlier, to use chemical propulsion to send a spaceship to Mars. In von Braun's proposal, Stuhlinger noted that the ratio of take-off weight to final weight after propellant consumption was 25-to-1. Stuhlinger argued that lighter-weight electric propulsion systems would make such planetary trips more feasible than they were with chemical propulsion. In April of 1958 Army Ballistic Missile Agency in Huntsville, Alabama initiated its first electrical propulsion contract.
In 1960’s there are major advances in ion propulsion included multi-aperture grids, mercury vaporizers, long-life oxide main cathodes, plasma bridge neutralizers, and discharge chamber hollow cathodes. The major development programs included 5, 10, 20, 50 and 150 cm thrusters. In 1961 (August) Hughes Research Laboratory in Malibu, California, under contract with the Marshall Space Flight Center in Huntsville, Alabama, demonstrated an ion engine. In December of 1962 Program 661A Test Code A – The first of three sub-orbital flight tests was launched of the Electric Propulsion Space Tests. Engine thrusting was not accomplished in this test. In July 1964, the Space Electric Rocket Test (SERT I) spacecraft was launched using a Scout launch vehicle. This flight experiment had an 8-cm-diameter cesium contact ion engine and a 10-cm-diameter mercury electron bombardment ion engine and was the first successful flight test of ion propulsion. One year later a SNAP 10 A nuclear power system was launched into a 1300-km orbit with a cesium ion engine as a secondary payload. Three years later two caesium-contact ion engines were launched aboard the ATS-4 spacecraft. This was the first successful orbital test of an ion engine. The same year ion propulsion was mentioned in Star Trek episode “Spock’s Brain.”
In February 1970, the SERT II spacecraft was launched into a 1000-km-high polar orbit. In addition to diagnostic equipment and related ion 5 propulsion system hardware, the spacecraft had two identical 15 cm diameter, mercury ion engines and two power-processing units. In May 1974 the ATS-6 was launched. One of the ion engines operated for about one hour and the other for 92 hours. Three years later ion propulsion was used again as science fiction on the Twin Ion Engine (TIE) fighters (Star Wars movie).
In 1990’s Jet Propulsion Laboratory and NASA Lewis partnered on the NASA Solar Electric Power Technology Applications Readiness (NSTAR) project. In 1997 (September) NSTAR test concluded after over 8,000 hours of operation. The next year Deep space 1 was launched. DS1 is the first spacecraft to use ion propulsion to reach another planetary body. Deep Space 1 tested all these advanced technologies together so that other missions wouldn't have to bear the costs of being first. The mission concluded three years later in 2001, after approaching two asteroids. The mission achieved a specific impulse of 1000-3000 seconds which is higher than the specific impulse of chemical spaceships by a factor of 10, and a Thrust of 92 milli-Newtons. Thrust is of course proportional to the acceleration of the spaceship, and ‘specific impulse’ is a special terminology which is used in rocket science. It can be defined as the impulse given to a spacecraft per unit weight of propellant used. It is dimensionally equivalent to the generated thrust divided by the propellant mass flow rate or weight flow rate. If mass is used as the unit of propellant, then specific impulse has units of velocity. If weight is used instead, then specific impulse has units of time. The better the propellant, the higher is the specific impulse. The engine worked for 678 days, a record at the time, which now belongs to the mission ‘Dawn’. NASA's Jet Propulsion Laboratory decided to test the function of the NSTAR thruster more extensively- a flight spare thruster identical to one flown on the successful Deep Space 1 mission. This test was concluded on June 30, 2003, after 30,352 continuous hours of operation.
In 2001 NASA's Office of Space Science selected Glenn Research Center to develop a next generation ion propulsion system called NASA's Evolutionary Xenon Thruster (NEXT) system. In 2003 at the end of NEXT Phase 1, NASA's Glenn Research Center (GRC) had successfully demonstrated system level performance of an engineering model thruster, a power processing unit and a propellant management system at power levels in excess of 7.0 kW.
Asteroid 1992 KD is also known as Asteroid Braille. On July 29, 1999 the spacecraft flew 26 kilometres away of the asteroid. The spacecraft's infrared sensor confirmed that the small asteroid is similar to Vesta , Braille was discovered in 1992. In April 2004 43 ignitions and over 6000 hours have been accumulated on a single unit of the Plasma Contactor Unit (PCU) which was developed by the Rocket dyne division of the Boeing Company to control charging of the International Space Station (ISS).
In September 2007 Dawn spacecraft was launched. This is the first purely scientific mission to use ion propulsion.The Dawn spacecraft uses ion propulsion to get the additional velocity needed to reach Vesta once it leaves the Delta rocket. It also uses ion propulsion to spiral to lower altitudes on Vesta, to leave Vesta and cruise to Ceres and to spiral to a low altitude orbit at Ceres. Ion propulsion makes efficient use of the on board fuel by accelerating it to a velocity ten times that of chemical rockets. This efficiency is measured in terms of the specific impulse of the fuel (Isp). Dawn's engines have a specific impulse of 3100 s. While a chemical rocket on a spacecraft might have a thrust of up to 500 Newtons, Dawn's much smaller engine achieves an equivalent trajectory change by firing over a much longer period of time.
There are several advantages of Xenon. The ion propulsion system uses electrical power to ionize and accelerate the propellant. The action of the ions leaving the thruster causes a reaction that pushes the spacecraft in the other direction. Although this system is extremely efficient, the thrust is very low. But the more massive the ion, the greater the thrust. Xenon, being a relatively massive atom, yields a higher (but still low!) thrust than many other candidate propellants. It is the most massive nonradioactive noble gas (outweighing helium, neon, argon, and krypton). Being nontoxic, it is easy and safe for engineers to work with. Because it is inert, the xenon atoms and ions that happen to make their way to sensitive spacecraft surfaces do not react chemically to degrade thermal, electrical, or optical properties. The xenon is relatively easy to store on board with conventional high pressure systems at typical spacecraft temperatures. The companies extract Xe from the atmosphere. When atmospheric nitrogen is liquefied, it contains a mixture of noble gases which are subsequently separated. Because there is such an enormous industry associated with nitrogen, the production of purified xenon is not as expensive as it would otherwise be.
It seems therefore that after the successful mission of Deep Space 1, ion propulsion will be the future of space exploration. This is not surprising at all after all. Ion engines can eject ions over long periods of time and accumulate higher acceleration over time compared with a conventional rocket. As a matter of fact their specific impulse is very high.
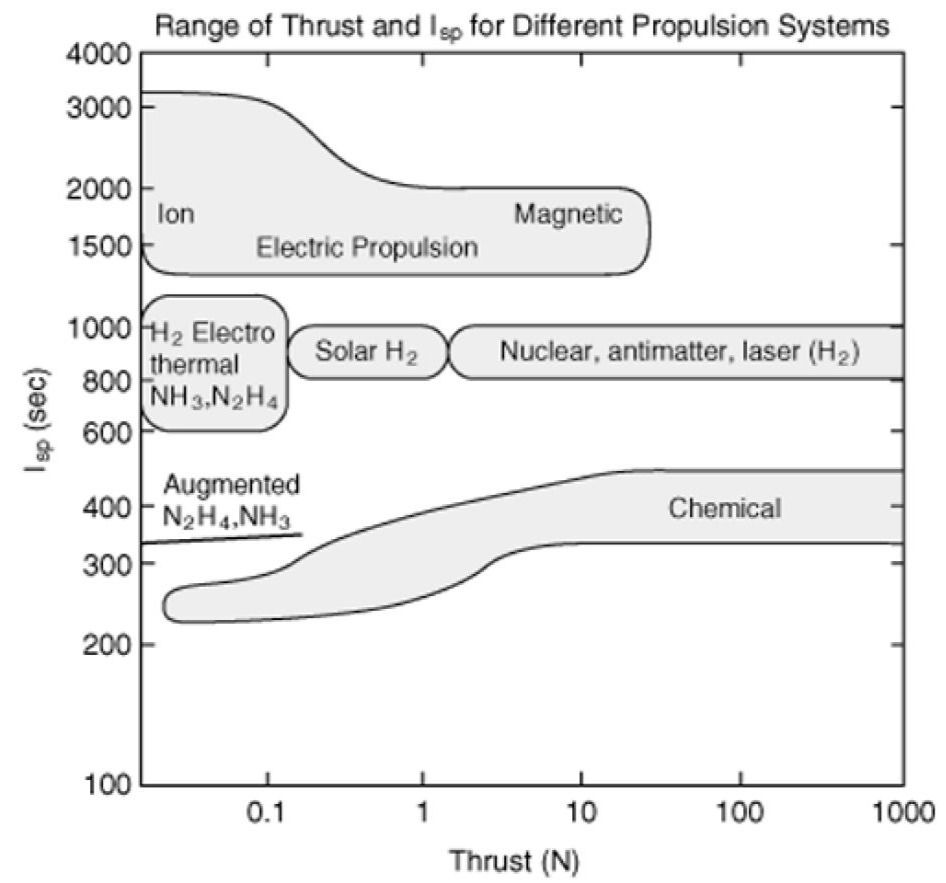
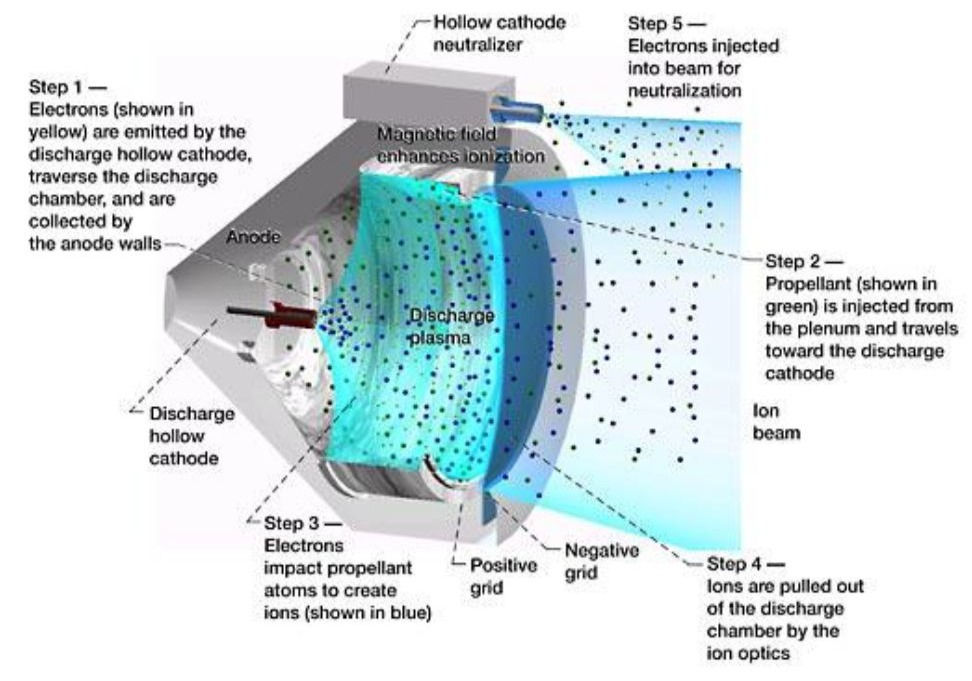
An ion engine accelerates ions using a voltage difference. Propellant (such as Xenon) enters from the left. A cathode emits electrons towards the same direction which knock the Xenon atoms, which in turn loose an electron and so positively charged xenon ions are created. These ions are then forced by gas pressure through holes in the positive grid. Then the electric field between the positive and negative grids accelerates the ions and sprays them out the back. The beam is neutralised by electrons. This happens in order to avoid the attraction of ions back to the negative grid, cancelling out the thrust. By ejecting electrons to the exhaust, a neutralisation of both the exhaust and the spaceship is achieved. Electrons carry little momentum and thus thrust is not affected. It is comprised of neutral gas molecules. There is very small radial field across the chamber, and the cathode can be a thermionic emitter. The electrons as they are accelerated, achieve typically energies of several tens of electron volts, which suffice to ionise the neutral Xenon atoms, by knocking them. To increase the path length of the electrons and ensure that they collide with as many Xenon atoms as possible, an axial magnetic field is generated, which forces them to move in a spiral path. This way ionisation becomes as much efficient as possible. In other words, the number of ions created related with the electron current, becomes maximum. The grids are separated by 1-2 mm, and a large potential differences, usually of order of 1000 volts. The ions gain energy in the strong electric field, and directed through the outer grid, they form the exhaust beam. Furthermore, in opposition with chemical rockets, a nozzle is not needed, since the motion of the ion beam, is very ordered and not chaotic, as in the case of chemical rockets. The thrust is exerted upon the grids, the grid is therefore transmitted to the whole body of the spacecraft.
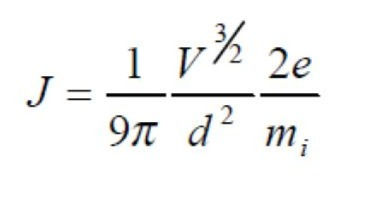
The equation above demonstrates how the current density J, as well as thrust depend only on the accelerating potential V and the grid distance d to each other, if enough ions can be created. If we increase the accelerating potential or decrease the grid separation the thrust can be increased, however, electrical breakdown between the grids puts an upper limit on this. Space charge limit sets an upper limit on thrust density to some Newtons/m^2 and so to generate higher thrusts, a larger thruster diameter is required. NASA’s Deep Space 1 was powered by a 30 cm diameter, 2 kW electron bombardment ion thruster having a specific Impulse of 3100 s. It achieved a beam current of 1.76 amps with a thrust of 92 mN but consumed only 12 kg of propellant during its entire mission. An advantage of ion thrusters is that a higher fraction of the input power is transmitted into kinetic energy of the exhaust than in a chemical rocket, because chemical rockets work as heat engines obeying the restrictive laws of thermodynamics.
Ion propulsion Subsystems
Ion propulsion systems comprise of the following five parts:
Power Source
Power processing unit
Propellant management system
Control Computer
Ion Thruster
Low thrust Devices
Except from ion thrusters there are also other categories of low thrust devices. These are:
1. Electrothermal devices, in these thrusters the exhaust is heated by some electrical process, then expanded through a suitable nozzle. Three sub-classes exist and these are different, in terms of the physical details of the propellant heating:
Resistojets, devices where the heat is transmitted to the fuel from a solid surface, (i.e. a chamber wall)
Arcjets, devices where the fuel is heated by an electric arc passing through it. An electric arc is an electric current, often strong, and luminous, in which electrons jump across a gap.
Inductively and radiatively heated devices. High frequency radiation heats the fuel.
2. Electrostatic devices, there the exhaust is accelerated by direct application of electrostatic forces to ionized particles.Ion thrusters are in this category.
3. Electromagnetic devices. In these devices the fuel is accelerated under the simultaneous action of electric and magnetic fields.Following decades of scientific and technological research, there is rapid development since the decade of 1950s, and a large number of variations in the operation of electromagnetic thrusters, have been constructed. Possible permutations of the electromagnetic thrusters have been studied, both experimentally and theoretically, but through the years only a few of them, survived. It is worth mentioning, the most advanced of them:
The steady or quasi-steady magnetoplasmadynamic (MPD) thrusters
The Hall-current accelerators,
The pulsed plasma devices.
4.Space sails, which use radiation pressure from the Sun ,to accelerate. That is the use the pressure of photons and not the pressure of solar wind. Sunlight at a distance of 1 AU(150,000,000 km) exerts a force of 9 Newtons per square kilometer.
5.Beamed energy devices, which use a remote energy source, such as a ground or space based laser or microwave source, to receive power from, via a beam of electromagnetic radiation.
Ion propulsion is clearly, a huge improvement in space exploration, and in the following years, it will improve significantly. Despite their very low thrust, ion thrusters today are clearly, the thrusters, with the highest specific impulse. The space charge limit, allows a maximum efficient current that can pass through the grids, limiting this way the thrust they can produce. Their advantage over conventional rockets is that they can operate for months accumulating acceleration and speed. We conclude that in principle they can be used successfully for interstellar travel, because of their economic fuel requirements.
Comentários